Remediating Fukushima—“When Everything Goes to Hell, You Go Back to Basics”
ENERGY, 14 May 2018
William Steel | Ars Technica – TRANSCEND Media Service
It may take 40 years for the site to appear like “a normal reactor at the end of its life.”
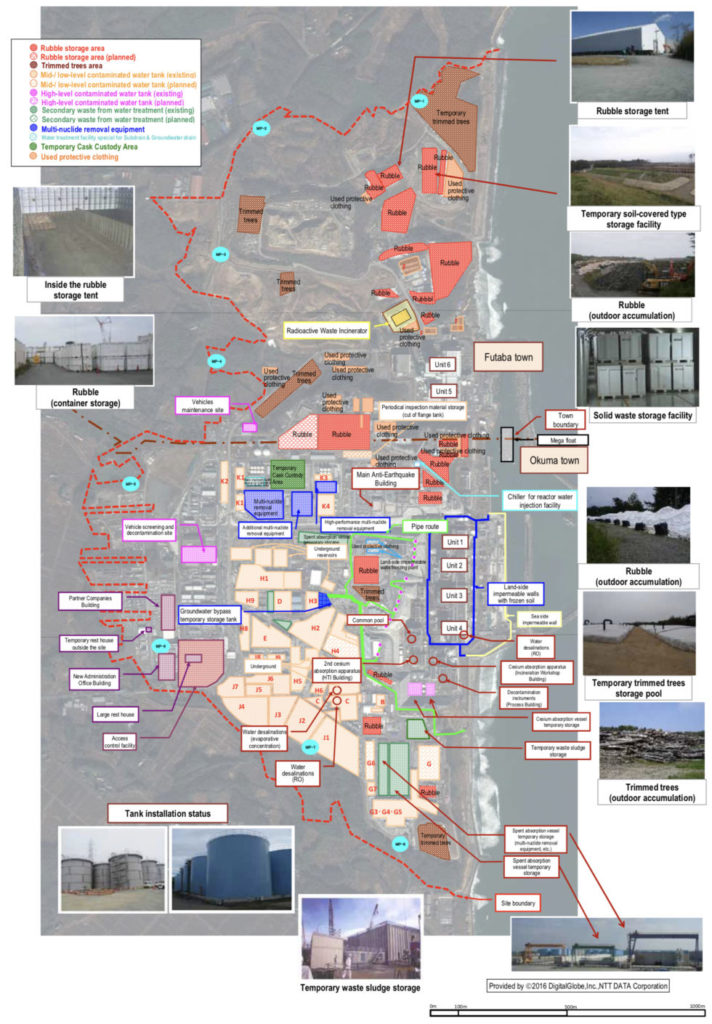
A schematic of the Fukushima nuclear power plant hints at the complexity of decontamination and decommissioning operations. TEPCO
11 May 2018 – Seven years on from the Great East Japan Earthquake of March 2011, Fukushima Daiichi nuclear power plant has come a long way from the state it was reduced to. Once front and center in the global media as a catastrophe on par with Chernobyl, the plant stands today as the site of one of the world’s most complex and expensive engineering projects.
Beyond the earthquake itself, a well understood series of events and external factors contributed to the meltdown of three of Fukushima’s six reactors, an incident that has been characterized by nuclear authorities as the world’s second worst nuclear power accident only after Chernobyl. It’s a label that warrants context, given the scale, complexity, and expense of the decontamination and decommissioning of the plant.
How does a plant and its engineers move on from such devastation? The recovery initiatives have faced major challenges, constantly being confronted by issues involving radioactive contamination of everything from dust to groundwater. And those smaller issues ultimately complicate the remediation effort’s long-term goal: to locate and remove the nuclear fuel that was in the reactors.
A Sense of Scale
Jonathan Cobb, spokesperson for the World Nuclear Association, spoke with Ars about the scale of Fukushima, explaining that radioactive releases in Japan were much smaller than at Chernobyl, and the accident resulted in no loss of life from radiation: “Of course, this doesn’t take away from the enormous task currently being faced at Fukushima.”
The UN Scientific Committee on the Effects of Atomic Radiation (UNSCEAR) reported in May 2013 that radiation exposure following the Fukushima accident didn’t cause any immediate health effects and that future health effects attributable to the accident among either the general public or the vast majority of workers are unlikely. A 2017 paper from UNSCEAR reports that these conclusions remain valid in light of continued research since the incident.
Even the most at-risk citizens, those living in Fukushima prefecture, are only expected to be exposed to around 10mSv as a result of the accident over their lifetimes. “For reference, the global average natural background radiation tends to be around 2.4mSv/year, but even 20mSv/year isn’t exceptional,” said Cobb.
Still, the accident was rated a 7 on the International Nuclear and Radiological Event Scale (INES), which is the highest rating possible, and designates it a Major Accident due to high radioactive releases. Estimates vary slightly, but Japan’s Nuclear Safety Commission report puts total releases at 570 petabecquerels (PBq) iodine-131 equivalent. (For comparison, Chernobyl released 5,200PBq iodine-131 equivalent.)
But the severity of the accident is probably most keenly felt in the scale of the cleanup. The incident has necessitated the ongoing cleanup and decommissioning of the plant—something that Tokyo Electric Power Company (TEPCO), the plant’s owner and operator, is responsible for. Even though the plant is seven years into the cleanup and has accomplished a great deal, we won’t see a conclusion for decades yet.
Meltdowns and Immediate Priorities
Remarkably, seismic shocks of the magnitude 9 earthquake didn’t cause any significant damage to the earthquake-proofed reactors; rather, the tsunami knocked out power that precipitated reactor meltdowns in Units 1, 2, and 3. Subsequent explosions caused by hydrogen buildup (from zirconium cladding of fuel assemblies melting and oxidizing) in Units 1, 3, and 4 then expelled radioactive contamination, most of which fell within the confines of the plant.
Cobb explained that in the aftermath of this, the ongoing risk posed by radionuclides (notably, iodine-131 and cesium isotopes 134 and 137) depended on their half-lives. Iodine-131, with a half-life of just eight days, posed virtually no threat at all after just several months. It has been cesium-134, with a two-year half-life, and cesium-137, with a 30-year half-life, that have been the major focus of decontamination efforts. “Radioactive decay means that we’ve seen a reduction in contamination simply through time passing; at the plant, however, my expectation is that the majority of reduction has been due to efforts of TEPCO. Conditions have improved markedly and a sense of normalcy has returned.”
It’s useful to take stock of what TEPCO had to contend with from the outset. Lake Barrett, a veteran of the US nuclear energy industry who spent several years at the helm of decommissioning work at Three Mile Island reactor 2, is currently an independent special advisor to the Japanese Government and TEPCO board of directors. He told Ars, “When everything goes to hell on you, you go back to basics. You’re concerned with accident response and immediate recovery of the situation. Over the longer timeframe, the decontamination & decommissioning (D&D) focus shifts to a more deliberate approach to major technical challenges.”
Barrett explained that reactor stabilization at Fukushima—an imperative of the immediate recovery—has long since been achieved. Temperatures within the Reactor Pressure Vessels (RPVs) and Primary Containment Vessels (PCVs) of Units 1-3 are stable at between 15 to 30ºC, and there have been no significant changes in airborne radioactive materials released from reactor buildings. This qualifies as a ‘comprehensive cold shutdown’ condition.
Barrett explained how the issue of cooling is mostly non-existent at this point: “The three melted reactor cores emit less heat than a small car. Decay heat was a huge issue in the first weeks, but it’s no longer an issue. And while TEPCO still injects water onto the cores, this is more for dust suppression than anything else.”
With the reactors stable, early phases of TEPCO’s work simply involved debris clearing and restorative efforts throughout buildings and across the 3.5 square miles of the plant—both having been ravaged by the earthquake and tsunami. In the most contaminated places, remotely operated machines undertook most of the work. To reduce environmental contamination, they also removed top soils and vegetation, deforested the site, and then applied a polymer resin and concrete across much of the plant complex. This has locked contaminated material in place and limited the flow of groundwater through the site.
Other work has been more substantial. Units 1, 3 and 4 were blown apart and have had to be reinforced and encased, both for safety and to prevent spread of radioactive material. Although Unit 2 retained its roof, TEPCO decided to dismantle the upper building nonetheless, as it will facilitate removal of fuel from the reactor.
At the peak of these operations, some 7,450 persons worked at Fukushima. As operations have evolved, the workforce has declined to a not inconsiderable 5,000 daily personnel. With such levels of permanent staffing, it’s little wonder that a new rest-house, cafeteria, shops, and office building have all been built.
The efforts have, in a practical sense, meant that the majority of the site has transitioned to a stable, relatively risk-free environment. Describing the decommissioning as an “enormous challenge never before undertaken by humanity,” Seto Kohta of TEPCO told Ars: “We have overcome the state of chaos that ensued after the accident and have succeeded in reducing site dose levels to an average of less than 5μSv/h, with the exception of the vicinity of Units 1-4.” (Global background levels are <0.5µSv/h.)
TEPCO reports that the additional effective dose (i.e. additional to natural background radiation) at the plant’s boundary has declined to the target value of less than 1mSv/y.
This is not to say the plant is without signs of past problems—far from it. Felled trees sit waiting for incineration; huge mounds of soil lie under tarps; buildings retain marks of past trauma; and with environmental dosage a perennial concern, close to a hundred dose-rate monitors are positioned around the site.
Kohta also noted that while “95 percent of the site no longer requires the donning of full- or half-face masks or coveralls,” some level of protection is still required for working around the plant according to three levels of contamination. The vast majority of the plant grounds are in what’s termed Zone G, which requires just generic coveralls and disposable medical masks. Zone Y provides a perimeter around the Units 1-4 and necessitates heavier-duty coveralls and either full- or half-face masks. And lastly there is Zone R, closer to and including the reactor buildings, requiring double-layered coveralls and full-face masks.
Reactor Investigations
While they’re now stable in terms of nuclear activity, Units 1-3 remain highly contaminated. As such, while the structural integrity of these buildings has been restored, relatively little work has been undertaken within them. (One notable exception is removal of contaminated water from condensers, completed last year.)
Over recent years, a variety of remotely operated devices and imaging technologies have performed investigations of these units. The intention has been to gather information on internal physical and radiological conditions of the PCVs—the heavily reinforced bell-shaped structures that host reactors. TEPCO wants, and needs, to understand what has happened inside. Some things are known: once melted, fuel mixed with structural materials including steel and concrete to form something known as corium. But precisely where the corium ended up, how much there is, and whether it’s submerged are just some of the questions in play.
The International Research Institute for Nuclear Decommissioning (IRID), which was established in April 2013 to guide R&D of technologies required for reactor defueling and decommissioning, is supporting TEPCO in seeking answers. IRID is composed of multiple stakeholders, including Japanese utilities and the major nuclear vendors Hitachi, Mitsubishi, and Toshiba.
Naoaki Okuzumi, senior manager at IRID, described for Ars the investigative approaches and technologies. Early work utilized Muon tomography, which Okuzumi described as “a kind of standard practice applied to each unit… to locate high density material (fuel) within PCVs.” It yielded low-resolution data on the approximate location of corium. But with pixels representing 25cm-square cross-sections, the information has been useful only in so far as validating computational models and guiding subsequent robotic investigations.
The latter task hasn’t been easy. In addition to the challenge of navigating the dark, cramped labyrinths of tangled wreckage left behind, TEPCO has had to contend with radioactivity—the high levels act something like noise in electronic circuits. The wreckage has made access a challenge, too, although varying points of ingress have been established for each PCV.
The circumstances mean that TEPCO hasn’t been able to simply purchase an off-the-shelf kit for these investigations. ”An adaptive approach is required because the situation of each PCV is different… there is no standard with investigating the PCVs by using robots,” said Okuzumi, describing an approach that has translated into devices being specially developed and built in response to conditions of each PCV.
But they’re making progress. As recently as January 2018, corium was identified for the first time inside Unit 2 using an enhanced 13m-long telescopic probe and a revised approach designed to overcome problems encountered during investigations in 2017. The situation was hardly easier at Unit 3, where the PCV is flooded to a depth of around 6.5m. Here, it took a remotely operated, radiation-shielded submersible called ‘Little Sunfish’ to locate corium in July 2017.
Altogether the investigations—featuring a litany of robotic devices—have revealed that little fuel remains in any of the cores of Units 1-3. In Unit 2, a large amount of corium is present at the bottom of the RPV; in Units 1 and 3, almost all fuel appears to have melted through the RPVs entirely and into the concrete floor of PCVs beneath. The information is crucial, as we’ll come to see, for future deconstruction work at the reactors, but it continues to be extended as investigations continue.
httpv://www.youtube.com/watch?v=infVQiVgW1k
PCV investigations at Unit 2
Pumps, Ice-Walls, and Storage: Water Management
One of TEPCO’s major concerns has been groundwater, which runs down from mountains west of the plant and can become contaminated by the low-lying reactors before flowing out to sea. Groundwater management has subsequently become one of TEPCO’s greatest efforts, as well as one of the most challenging of the tasks it has faced.
First off, it ought to be noted that marine environment monitoring for radionuclide concentrations near the plant and as far away as Tokyo indicate that levels are well within WHO standards. “The levels of radioactivity that have been found and can be attributed to Fukushima are absolutely dwarfed by natural levels of radioactivity in the water, or even levels of cesium that came from historic nuclear weapons testing,” noted Cobb.
Still, the effort to limit further contamination—seemingly driven as much by societal-political dynamics as safety considerations—remains paramount. To this end, measures have been deployed along three principles: remove sources of contamination, isolate water from contamination, and prevent leakage of contaminated water.
Some measures have been simple enough in design. Installation of an impermeable, underground wall along the sea front, completed in October 2015, is intended to keep groundwater that passes Units 1-4 from reaching the sea. Waterproofing pavement against rainwater is another widely applied step.
After this, solutions become more sophisticated. A groundwater bypass that intercepts and pumps up water before it reaches the reactors is a key development. This water is inspected for contamination before being discharged into the sea. By November 2017, more than 337,000 cubic meters of water had been released to the ocean in this way; this bypass reduced the amount flowing into the building basements by up to 100 tons per day and successfully reduced groundwater levels around the reactor buildings.
To further limit groundwater flow into reactors buildings, TEPCO actually froze the ground around them, creating a kind of frozen wall down to a depth of about 30 meters. Approximately 1,500 meters long, the wall is kept frozen by pipes filled with an aqueous solution of calcium chloride cooled to -30ºC. Freezing commenced in March 2016 and is now “99 percent complete,” according to Kohta.
On either side of the frozen wall, sub-drains and groundwater drains have been installed; they pump water up to keep it from reactor buildings and reaching the sea, respectively. Pumped water is purified at a purpose-built treatment facility. Barrett commented: “With water released from sub-drains and the bypass, there’s an agreement with the fishing industry that releases must be below 1,500 becquerels per liter. Negotiations took several years to agree that level was ‘clean’.”
All this has come at enormous expense, but according to TEPCO, it has been successful. Before any measures were implemented, inflow was around 400m3/day, Kohta told Ars. “The average amount of water flowing into [Units 1-4] for the period from December 2015 to February 2018, before the closure of the land-side impermeable wall, was 190m3/day, and it has decreased to 90m3/day after the closure for December 2017 to February 2018.”
At face value, it’s a sound outcome. As Kohta noted, the amount of contaminated water now being generated—a mix of groundwater, rainwater and water pumped into reactors for cooling—has decreased from about 520m3/day to about 140m3/day between last December and February. Even so, treating that amount of contaminated water is proving taxing.
Water treatment is happening at large-scale facilities that have been built onsite, including a multi-nuclide removal facility. Here, a so-called Advanced Liquid Processing System (ALPS) reduces concentrations of cesium isotopes, strontium, and other radionuclides to below legal limits for release. But one radionuclide remains: tritium.
Cobb explained: “The difficulty is that tritium is basically an isotope chemically identical to hydrogen, so it’s impractical to remove. Levels of tritium in that water are low, but nevertheless there’s great sensitivity to the suggestion that it be discharged.”
Without a feasible alternative for cleaning up the tritium, the (only) solution for ALPS-treated water has been storage. Well over a thousand tanks, each holding 1,200 cubic meters, now store tritium-laced water at the south end of the plant. Several years ago, these tanks hit the news because several were found to be leaking. Barrett acknowledged it as an unfortunate and avoidable incident resulting from use of flange-tanks. TEPCO has since moved to more sturdy welded-joint water storage tanks.
The ultimate plan for stored water is unknown; tritium has a half life of a dozen years, so physics won’t clean up the water for us. Some kind of controlled, monitored discharge—the likes of which is typical within the nuclear industry—is possible, according to Barrett. Indeed, the International Atomic Energy Agency has endorsed such a plan, which was proposed by the Atomic Energy Society of Japan in 2013. The plan involved diluting tritiated water with seawater before releasing it at the legal discharge concentration of 0.06MBq/L and monitoring to ensure that normal background tritium levels of 10Bq/L aren’t exceeded.
Discussions at both national and international levels would need to come first. Part of the difficulty here harkens back to societal dynamics surrounding risk and contamination: “In nuclear there is no such thing as absolute zero—sensitivity goes down to the atom. This makes discussion about decontamination or levels of acceptable contamination difficult. There’s tritium in that water that’s traceable to the accident; it’s entirely safe, but for the time being, with the event still in recent memory, it’s not acceptable,” observed Barrett.
Toward Permanent Solutions
In some sense, much of the restoration of order at Fukushima has been superficial—necessary but concerned with handling consequences more than root causes (see, TEPCO interactive timeline). Ultimately, Fukushima’s reactors must be decommissioned. Broadly, this work involves three phases: removing used fuel assemblies that are stored within ten-meter-deep spent fuel pools of each reactor building, management of melted-down reactors and removal of corium debris, and deconstruction of reactor buildings and the greater plant.
At Unit 4, spent fuel removal operations took around 13 months and concluded in December 2014. “When we began we didn’t know if fuel assemblies or racks were distorted. It turned out they weren’t, and we were able to remove all fuel conventionally without any issues at all. Actually, it went exceedingly well, concluding ahead of schedule and under cost,” recalled Barrett. In all, 1,533 fuel assemblies were removed and transferred to a common spent fuel pool onsite.
httpv://www.youtube.com/watch?v=bk7e2MHxh2Y
Spent fuel removal at Unit 4 was accomplished with conventional techniques.
Defueling of pools at Units 1 through 3, which suffered meltdowns, isn’t going to be as straightforward. For one, there’s some expectation of debris and circumstances requiring extraordinary removal procedures. “I wouldn’t be surprised if we find some structurally bent fuel assemblies caused by large pieces of concrete or steel,” said Barrett. Additionally, although radiation in Unit 3 has been reduced sufficiently to allow rotating shifts of workers to install defueling equipment, the already painstaking operations will have to be conducted remotely. The same is likely true for Units 1 and 2.
At Unit 3, the next in line for defueling, preparation is already well underway. In addition to decontamination and installation of shielding plates, TEPCO has removed the original fuel handling crane, which had fallen into the pool seven years ago, and installed a new fuel handling crane and machine. An indication of extraordinary containment methods being used, workers have built a domed containment roof at Unit 3. TEPCO’s Kohta told Ars, “Removal of spent fuel [at Unit 3] is scheduled to begin from around the middle of 2018;” meanwhile, Unit 1 is also in a preparatory stage and Unit 2 will be handled last.
Further down the line still, corium will have to be removed from melted-down reactors. It’s a daunting task, the likes of which has never been undertaken before. The reactors held varying, but known, amounts of uranium oxide fuel, about 150 tonnes each. But how much extra mass the fuel collected as it melted through reactor vessels is uncertain.
“At TMI there was exactly 93 tonnes in the reactor. Once we were done digging out fuel debris, we’d removed 130 tonnes. At Fukushima, I expect maybe a factor of five to ten more mass in core debris. It’s an ugly, ugly mess underneath the PCVs,” suggested Barrett.
High-powered lasers, drills and core boring technologies for cutting, and strong robotic arms for grappling and removing corium are already under development, according to IRID, but precise methodologies remain undecided.
The original plan, Barrett explained, was to flood PCVs and work underwater—a conventional nuclear operations technique that affords protection from contamination. But this requires water-tight PCVs, something that cannot be practically achieved at Fukushima. Discussions also continue over whether a side or top-down entry would be best. “Altogether, we don’t have enough physical data about PCVs to commit to a final decision,” said Barrett, referring back to the need for continued PCV investigations. According to Kohta, fuel debris removal isn’t scheduled to commence before the end of 2021.
Without doubt, the road ahead of TEPCO is a long one, beset with challenges greater than those faced to date. The Mid- and Long-Term Roadmap—the Japanese state-curated document outlining the decommissioning of Fukushima—envisions operations stretching a full 30-40 years into the future. Some have suggested it’s an optimistic target, others say that the plan lacks details on key, long-term issues such as permanent solid-waste storage beyond the onsite repository currently being employed. Certainly it is the case that key decisions remain.
For his part, Barrett concluded: “I believe that the 40-year timeframe is reasonable for a scientifically based decommissioning; that’s to say, to reach a point similar to that of a normal reactor at the end of its life. That’s not reaching the point of a green field where you’d want to put a children’s school. Could it be a brown-field, industrial site, though? Yes it could. That’s a rational, reasonable end point.”
By all accounts, it is hard to gauge the costs for the Fukushima clean-up. Kohta told Ars that works completed to date have cost about 500.2 billion yen, or $4.7 billion—a tremendous sum, to be sure, but fractional compared to the estimate of 8 trillion yen ($74.6 billion) approved by the Japanese state last May for the complete decommissioning of Fukushima Daiichi.
Go to Original – arstechnica.com
DISCLAIMER: The statements, views and opinions expressed in pieces republished here are solely those of the authors and do not necessarily represent those of TMS. In accordance with title 17 U.S.C. section 107, this material is distributed without profit to those who have expressed a prior interest in receiving the included information for research and educational purposes. TMS has no affiliation whatsoever with the originator of this article nor is TMS endorsed or sponsored by the originator. “GO TO ORIGINAL” links are provided as a convenience to our readers and allow for verification of authenticity. However, as originating pages are often updated by their originating host sites, the versions posted may not match the versions our readers view when clicking the “GO TO ORIGINAL” links. This site contains copyrighted material the use of which has not always been specifically authorized by the copyright owner. We are making such material available in our efforts to advance understanding of environmental, political, human rights, economic, democracy, scientific, and social justice issues, etc. We believe this constitutes a ‘fair use’ of any such copyrighted material as provided for in section 107 of the US Copyright Law. In accordance with Title 17 U.S.C. Section 107, the material on this site is distributed without profit to those who have expressed a prior interest in receiving the included information for research and educational purposes. For more information go to: http://www.law.cornell.edu/uscode/17/107.shtml. If you wish to use copyrighted material from this site for purposes of your own that go beyond ‘fair use’, you must obtain permission from the copyright owner.