“Green Energy”: Are Humanoids Safe?
TRANSCEND MEMBERS, 18 Oct 2021
Prof Hoosen Vawda – TRANSCEND Media Service
Death Knell for Planet Earth: Is Humankind Embracing a Greater Evil?
11 Oct 2021 – The Industrial Revolution[1], was a defining era in the history of Human Civilisation in the 18th Century, with the invention of the steam engine in 1712 by Thomas Newcomen[2], which formed the mechanical basis for massive industries emerging in Europe, the Americas, Asia and the Far East. Great factories were established to churn out daily common use items for the ever-increasing population of the world. These factories used fossil fuels[3], initially coal, then diesel and eventually electricity generated from coal burning power plants.
The discovery of oil in the Arabian Peninsula[4] on 31st May 1932, forever changed the eco-dynamics. not only of the Arab Block, for it generated immeasurable fortunes for the Arab States, principally, Saudi Arabia. This was reflected by the gift of Gold Rolex watches presented as gifts to all the staff of Omni Shoreham Hotel in Washington DC[5], when King Saud[6] visited the United States on an official visit after the discovery of crude oil, “Black Gold” in Arabia. This entity was then literally drained out from the bottom less oil wells by various American companies, who were given drilling rights by the Saudi Government at the time.
This oil discovery had an impact on the rapid expansion and growth on the automotive production industry, not only in North America, but even in Australia, Far East as well as in South Africa. The dependency on oil for energy continued until hydroelectric power stations were used to generate electricity. In Africa, the Russians built the Aswan Dam[7] on the River Nile where the 3000-year-old, ancient Pharaonic Temple at Abu Simbel[8] had to be relocated to a higher level, piece by piece, in view of the rising water levels of the Nile due to the building of Aswan Dam in 1960, to generate hydroelectricity.
In the Industrial Revolution 4.0[9] where Artificial Intelligence [10]“AI” dominates, there is a great enthusiasm on the promotion of “Green Energy”[11] to be in harmony with “Green Peace” movement by various mega corporations, companies and organisations. These attempts at reducing the emissions of Green House Gases[12] and diminishing carbon foot prints is borne out by engineering transformation in the field of utilising alternative energy sources, which do not use fossil fuels, is sustainable and does not pollute the environment. These are:-
- Fully, self-recharging electric vehicles[13]
- Wind Turbine Farms[14]
- Solar Farms[15]
- Megaprojects generating hydroelectricity[16]
In fact, China to build the world’s biggest dam on sacred Tibetan river, The dam on the Yarlung Tsangpo [17]is part of China’s bid to achieve carbon neutrality, but experts worry about the impact on the mighty river and lack of consultation with the affected people. While some of these Green Energy projects are in operation for over five decades, the renewed innovations in this field are cause for concern, especially when it comes to the extraction of raw materials and very rare minerals which are needed for the production of full electric vehicles. Although renewable energy sources produce relatively low levels of GHG emissions and conventional air pollution, manufacturing and transporting them will produce some emissions and pollutants. The production of some photovoltaic (PV) cells[18], used in solar panels, generates toxic substances that may contaminate water resources.
Mercedes Benz AG has “perfected” and launched its flagship fully electric vehicle, the EQS S Class Mercedes car[19], the first electric vehicle in the luxury class. The EQS is the first all-electric luxury saloon from Mercedes-EQ. With it, Mercedes-EQ is redefining this vehicle segment. The EQS is also the first model to be based on the modular architecture for luxury and executive-class electric vehicles. Fusing technology, design, functionality and connectivity, the EQS delights both drivers and passengers. First models on the market will be the EQS 450+ with 245 kW and the EQS 580 4MATIC with 385 kW. Designed to be sustainable. As part of its Ambition 2039 initiative, Mercedes-Benz is working on offering a carbon-neutral new car fleet within 20 years from now. By as early as 2030, the company wants more than half the cars it sells to feature electric drive systems – this includes fully electric vehicles and plug-in hybrids. In many areas, Mercedes-Benz is already thinking about tomorrow today: the new EQS is designed to be correspondingly sustainable. The vehicles are produced in a carbon-neutral manner, and resource-saving materials such as carpets made from recycled yarn are used. This is because Mercedes-Benz considers the entire value chain, from development and the supplier network to its own production methods. Mercedes-Benz AG has had its climate protection targets confirmed by the Science Based Targets Initiative (SBTI). The EQS S class is the most aerodynamic production car in the world. With a great deal of meticulous detail work and on the basis of the Purpose design, the aerodynamicists, in close cooperation with the designers, were able to achieve a new drag coefficient value, best value of 0.20. This makes the EQS the most aerodynamic production car in the world. The operating range particularly benefits from this. It is also among the best in terms of quiet running. The very low wind noise level contributes significantly to this. Furthermore, Intelligent energy recovery, ensures efficient usage. The EQS also currently sets a very good value for recuperation: of the maximum deceleration in the DAuto recuperation programme of 5 m/s, up to 3 m/s is achieved by recuperation (2 m/s by the wheel brakes). This allows decelerating to a standstill without using the brake pedal, while at the same time the range benefits from this recuperation strategy and the high recuperation power (up to 290 kW**). Deceleration is also applied to detected vehicles ahead until they come to a standstill, for example at traffic lights. Intelligent energy recovery is situation-optimised with the aid of ECO Assist and acts with foresight, considering traffic conditions or topography, among other things. The driver also can set three energy recovery levels and the coast function via paddle shifters on the steering wheel.
In addition, the Mercedes Benz EQS marks the launch of a new generation of batteries with significantly higher energy density. The larger of the two batteries has a usable energy content of 107.8 kWh. That is around 26 percent more than the EQC. The innovative battery management software, developed in-house, allows updates over the air (OTA). In this way, the energy management system remains up to date throughout the life cycle. In terms of the cell chemistry, the cobalt content of the cathodes has been reduced to ten percent. To optimize energy usage savings the vehicle has been subjected to a Rigorous Purpose Design philosophy. Although the EQS is a close relative of the new S-Class, it is built on an all-electric architecture. This completely new concept made a Rigorous Purpose Design possible: with its one-bow lines and cab-forward design with fastback, the EQS is clearly distinguishable from vehicles with combustion engines even at first glance. The design philosophy of Sensual Purity, combined with Progressive Luxury, is reflected in generously sculpted surfaces, reduced joints and seamless transitions (seamless design). The design picks up on the original star of the Daimler-Motorengesellschaft, which was registered as a trademark in 1911.
All this AI technological, advancement contributions and deployment ensure an extremely safe, Green Energy Vehicle, but let us examine the raw materials which go into the production of this ultimate in passenger vehicular contribution to Green Energy by Daimler AG and its vehicle division Mercedes-Benz AG.
The production of electric vehicles needs close scrutiny in view of the fact the large quantities of lithium[20] are needed for the “life giving” batteries for these vehicles. The production of electric vehicles (EVs) requires substantially larger quantities of minerals than internal combustion engine vehicles, such as copper[21]. Without these minerals, vehicle bodies and critical components such as engine magnets and batteries cannot be built. The rapid and continuing rise in EV production, as well as increasing use of many rare minerals in the production of other products such as aircrafts and consumer electronics, has focused attention on the availability of these metals. In all electric vehicles, the rare minerals needed a range from the widely recognisable aluminum, copper and silver, to the more esoteric cobalt, lithium and graphite.
Greater access to critical minerals will serve to ensure that the global community is able to achieve a future that is not entirely dependent on oil. At present, U.S. industry is vulnerable to supply chain disruptions precipitated by shortages of numerous minerals. Disruptions may arise through foreign government actions, disproportionate market power by a corporation, internal strife in producer countries, natural disasters, or other causes. In extreme cases, mineral supply disruptions could require extremely costly and sub-optimal substitution efforts or even force manufacturers to halt production.[22]
In an effort to ensure an adequate supply of critical minerals, the U.S. Department of the Interior (DOI) has recently intensified its efforts to work with industry and other stakeholders to avoid minerals shortages or supply disruptions. DOI’s first step has been to create a list of minerals deemed most “critical.” After this list is finalized, DOI will examine the various critical mineral supply chains to ascertain how U.S. policy can promote greater domestic production and enhance recycling and reprocessing. Ultimately, DOI will release a comprehensive plan with concrete steps to reduce this vulnerability. The positive news about this development is that smart public policy and redoubled industry supply chain management efforts should greatly ameliorate this growing strategic vulnerability. Presently, there is a fine balance of critical minerals in terms of supply and demand.
The primary threat to accessing automotive related critical minerals comes from foreign companies and countries restricting production for commercial or political reasons. With lithium, for example, China’s Tianqi Lithium is attempting to purchase a 32 percent stake in one of the world’s largest lithium producer (Chile’s Sociedad Quimica y Minera SA). If Tianqi succeeds in this bid, the two companies will control approximately 70 percent of global lithium supply. If this were to happen, Chinese battery manufacturers would have the ability to distort market prices and availability, and thus greatly reduce the competitiveness of American made batteries and EVs. Additionally, given the nature of China’s economic system, it is quite possible that if U.S.-China relations deteriorate sharply, Tianqi Lithium could be ordered by the Chinese government to manipulate the supply of lithium to advance Chinese state interests.
Increasing domestic exploration and production of critical minerals and promoting recycling and reprocessing are necessary to ensure automakers and technology developers have the resources they need to develop an advanced fuel vehicle transportation system.
A second threat to U.S. critical mineral access comes from global supply conditions and the lack of greater U.S. production and reprocessing facilities. Across the range of minerals, near-term and intermediate demand is expected to significantly outpace supply. Every EV needs magnets, microprocessors, and batteries, all of which are made with critical minerals. Some of the most important minerals include cobalt, graphite, lithium, manganese, and the rare earth elements dysprosium and neodymium. If one includes the demand for stationary power storage, wind turbines, cell phones, computers, and vehicle batteries, the consumption of these minerals could surge. In fact, Bloomberg New Energy Finance sees demand for Cobalt, for example, increasing as much as 30-fold between 2017 and 2030.
The global electric vehicle (EV) market should see considerable growth in the coming years. In 2017, approximately 197,000 EVs were sold in the United States. The U.S. Energy Information Administration projects EV sales to reach 1.4 million per year (or 7 percent of all light-duty vehicle sales) by 2025 and 10 percent of total sales by 2040. All of these vehicles require multiple minerals. Moreover, the United States will have to compete for the global supply of these minerals in a world where EV sales and consumer electronics also are rising sharply outside the country. The International Energy Agency estimates that annual global EV production will reach at least 40 million vehicles by 2040. Approximately, 73% of rare earth elements are used in mature industries, including glass, ceramics and metallurgy. The remaining 27% are used in the production of neomagnets, which are essential components in electric vehicles (EVs). Using the force produced when two magnets with opposing poles repel each other, electric motors use permanent magnets and coils that have been magnetised by electricity to propel an axle. The force (torque) of the spinning axle is used to power the wheels of an electric car. Induction-based electrical motors, which do not use permanent magnets, can also power EVs. However, they are an unpopular solution compared to their magnetic cousins. Tesla, one of the only holdouts in induction motors, has used a magnetic engine in its new Model 3. The rare earth elements used in EVs, principally go into magnet production. A magnet’s strength is commonly measured by its coercive force and flux density. Of the four main types of magnets, those made from the rare earth neodymium rate highest in coercivity, while retaining a similar flux density to their less powerful peers. This combination makes them perfect for high-powered EVs. The drawback of simple neodymium magnets is a low operating temperature. This means they lose their magnetism at temperatures of around 60–80°C, like those found in an electric engine. To mitigate this issue, the rare earth element dysprosium, or more rarely terbium, can be added, increasing operating temperatures to above 160°C.
Dysprosium, along with the rare earth element praseodymium, can also increase a magnet’s coercivity, when alloyed with neodymium. For this reason, neomagnets for EVs tend to be composed of around 24% neodymium, 7.5% dysprosium and 6% praseodymium.
The greatest density of rare earth projects is in Australia. Australian explorer Northern Minerals is set to open its pilot plant at the Browns Range, in its quest to become the largest producer of dysprosium outside China.
Elsewhere in Australia, Alkane Resources, under its fully owned subsidiary Australian Strategic Materials, has completed its feasibility study at Dubbo, in New South Wales. There is also action in Northern Australia, where Arafura Resources owns and operates the Nolans rare earth project on a large praseodymium deposit.
The Yangibana Rare Earth Project, operated by Hastings Technology Metals, has integrated nine mining licences in the Gascoyne region of Western Australia. Outside of Australia, Peak Resources owns the Ngualla Tanzania rare earth project, which expects to make 90% of its revenue from neodymium and praseodymium. And Rare Element Resources continues to operate the Bear Lodge Project, in Wyoming.
The International Energy Agency[23] (IEA) forecasts the EV fleet will grow from 3.1m in 2017 to 125m in 2030. Given a fully electric vehicle requires between 1kg to 2kg of neomagnets, with 0.42kg for hybrid variants, the market is set to expand massively over the next decade. Dysprosium demand for EVs in 2017 was around 180–360 tons. If the IEA’s forecast holds true, demand for dysprosium in EVs would reach 6,000–13,000t by 2030, while neodymium would go from 582–1,162t in 2017, to 20,000–40,000t by 2030. Praseodymium would grow from 150–300t in 2017 to 5,000–10,000t by 2030.
That said, the amount of rare earth elements required per EV is unlikely to remain stable, and will probably drop as the technology improves. Even so, a maturing EV industry represents an incredible growth in rare earth demand. Given China’s diminishing exports of rare earths, this demand may be difficult to meet over the medium term. China has dominated the supply chain for rare earths over the last 25 years, growing its market share from 54% in 1994 to highs of 97% in 2006. The virtual monopoly on supply led to price volatility in 2010, when China put export quotas on rare earths, driving the price upwards. The 2010 quotas led to a decline in global total rare earth oxide production of 30% in a single year. The quotas ceased in 2016, after the WTO ruled them to be unjustified, but China plans to continue its policy of reducing rare earth exports. This is part of its push towards environmental sustainability, high-tech markets and a consumer driven economy. The 2010 quotas artificially increased prices, with neodymium reaching peaks of $270/kg, dysprosium $1,600/kg and praseodymium $225/kg. Following the 2010 production shortage, prices declined between 2011 and 2015 to $48/kg for neodymium, $278/kg for dysprosium and $75/kg for praseodymium. Since then the price of dysprosium and neodymium has declined to $172/kg and $62/kg, respectively. Those weak price points have made investors wary of engaging in equity investment within the rare earth sector, with the entire prospect considered to be high-risk, low-reward. The growth of EVs, alongside the decline in Chinese production, will likely see prices increase over the medium term, making proactive investment into new projects more likely.
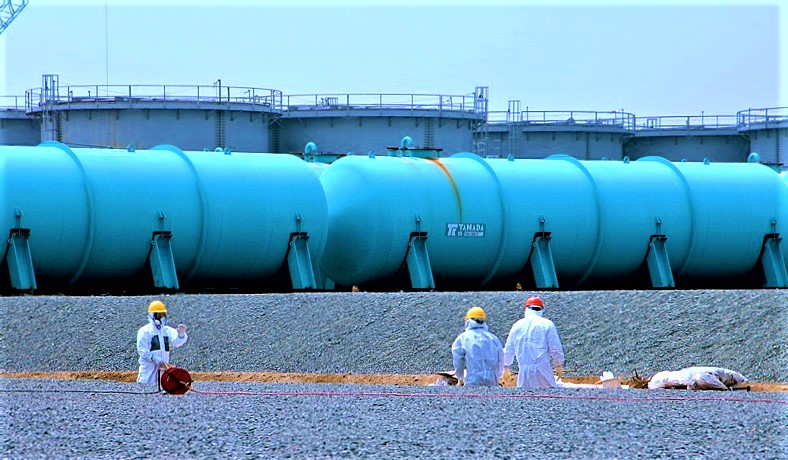
Two types of above ground water tanks and underground pool being stored in 1,000,000 gallon tanks – Fukushima Reactor in Japan
Just as presently, the problem of discarding of nuclear waste and nuclear contaminated water from the Fukushima[24] Reactor in Japan, being stored in 1,000,000 gallon tanks in a major challenge for the Japanese Government as well as environmentalists. By 2040, the recycling and disposal of lithium vehicle batteries would pose a major environmental hazard and will probably be worse than the present levels of industrial pollution, we have in the 21st Century. We are also unaware of medical hazards on the electroconductivity of the human heart, the effects of large vehicle lithium batteries on the human brains waves and the propensity to be the genesis of epilepsy or dementia would be a real threat to humankind in the future including the early development of senile dementias, psychiatric conditions, cardiac arrythmias and other degenerative brain condition, as well as the development of myopathies while sitting on top of and driving a vehicle which has large lithium batteries. The extraction and chemical processing of these rare minerals causes, massive environmental pollution and will certainly have an impact on the flora and fauna of a particular region, as well as affecting humanoids resident in that region This is borne out by the large scale, open mining of copper at Chuquicamata[25] in Chile. The copper is removed from site and transported to the port of Chile in open trucks for export. This has increased the incidence of lung cancers and chronic pulmonary diseases in the adjoining cities along the route of road transport of the copper ore as well as exposure and pollution of highly toxic slush from the processing sites being pumped into the adjoining rivers and lakes.
Similarly, the Atacama salt flat [26]is a majestic, high-altitude expanse of gradations of white and grey, peppered with red lagoons and ringed by towering volcanoes, in Northern Chile. The Atacama Desert experiences the Earth’s highest levels of solar radiation, and only parts of Antarctica are drier. In this environment there is an emerging environmental dilemma. In order to mitigate the accelerating climate crisis, humanity needs to rapidly reduce carbon emissions. Therefore, energy systems globally must transform from fossil fuels to renewable, Green Energy. Lithium batteries play a principal role in this transition as they power electric vehicles and store energy on renewable grids, helping to cut emissions from transportation and energy sectors. Only 130 feet below the Atacama salt flat lies most of the world’s lithium reserves. Chile currently supplies almost a quarter of the global market. However, extracting lithium from this unique landscape comes at a profound environmental and social cost.
In the mining installations, which occupy more than 78 sq km (30 sq miles) and are operated by multinationals SQM and Albemarle, brine is pumped to the surface and arrayed in evaporation ponds resulting in a lithium-rich concentrate; viewed from above, the pools are shades of chartreuse. The entire process uses enormous quantities of water in an already parched environment. As a result, freshwater is less accessible to the 18 indigenous Atacameño communities that live on the flat’s perimeter, and the habitats of species such as Andean flamingoes have been disrupted. This situation is exacerbated by climate breakdown-induced drought and the effects of extracting and processing copper, of which Chile is the world’s top producer. Compounding these environmental harms, the Chilean state has not always enforced indigenous people’s right to prior consent. The question which needs to be raised urgently, is that “will green technology kill Chile’s deserts?” The facts seen in te Attacama Desert raise an uncomfortable question which reverberates around the world. does fighting the climate crisis mean sacrificing communities and ecosystems? The supply chains that produce green technologies begin in extractive frontiers like the Atacama Desert. At present, we are on the verge of a global boom in mining linked to the energy transition. A recent report published by the International Energy Agency states that meeting the Paris agreement’s climate targets would send demand skyrocketing for the “critical minerals” used to produce Green Energy technologies. The figures are particularly alarming for the raw materials used to manufacture electric vehicles. It is estimated that by 2040, the IEA forecasts that demand for lithium will have increased 42 times relative to 2020 levels.
These rare and critical mineral resources have become a new flashpoint for geopolitical tensions. In the US and Europe, policymakers increasingly talk about a “race” to secure the minerals linked to energy transition and shore up domestic supplies. The idea of a “new cold war” with China is frequently invoked. As a result, northern Portugal and Nevada are slated for new lithium projects. Across the global lithium frontier, from Chile to the western United States and Portugal, environmental activists, indigenous communities and residents concerned about the threats to agricultural livelihoods are protesting over what they see as the greenwashing of destructive mining.
Indeed, natural resource sectors, which include extractive activities like mining, are responsible for 90% of biodiversity loss and more than half of carbon emissions. One report estimates that the mining sector produces 100bn tons of waste every year. Extraction and processing are typically water- and energy-intensive, and contaminate waterways and soil. Alongside these dramatic changes to the natural environment, mining is linked to human rights abuses, respiratory ailments, dispossession of indigenous territory and labour exploitation. Once the minerals are wrested from the ground, mining companies tend to accumulate profits and leave behind poverty and contamination. These profits only multiply along the vast supply chains that produce electric vehicles and solar panels. Access to these technologies is highly unequal, and the communities who suffer the harms of extraction are frequently denied its benefits. The Chilean activists are clear: there is no zero-sum conflict between fighting climate breakdown and preserving local environments and livelihoods. Indigenous communities in the Atacama Desert are also on the frontlines of the devastating impacts of global heating. Rather than an excuse to intensify mining, the accelerating climate crisis should be an impetus to transform the rapacious and environmentally harmful patterns of production and consumption that caused this crisis in the first place.
The second problem with Green Energy concerns the use of wind turbine farms to produce electricity. Apart from the danger to birds and bats, these wind turbines located on land could cause the widespread deforestation to accommodate the huge turbines. Which itself is an ecological disaster, furthermore when installed in seas, this metal of the turbines could cause disruption in aquatic ecosystems and destruction of marine life, including coral. In addition, the life span of these giant fans is only about 20 years and huge aluminum blades of the wind turbines are already littering the Netherlands countryside, could have a disastrous impact on the agricultural land as well as polluting the environment as the aluminum is too expensive to recycle and to discard the wind turbines at the end of their operative life span would be more economical.
Great human advertising energy and funds are spent on promoting solar power and creation of Solar Farms. These also require large, open spaces of land, to be installed. Often arable land is used, causing deforestation and radiation from the solar panels. Historically, in 1839, the ability of some materials to create an electrical charge from light exposure was first observed by Alexandre-Edmond Becquerel.[27] Though these initial solar panels were too inefficient for even simple electric devices they were used as an instrument to measure light. The observation by Becquerel was not replicated again until 1873, when Willoughby Smith discovered that the charge could be caused by light hitting selenium. After this discovery, William Grylls Adams and Richard Evans Day published “The action of light on selenium” in 1876, describing the experiment they used to replicate Smith’s results. In 1881, Charles Fritts created the first commercial solar panel, which was reported by Fritts as “continuous, constant and of considerable force not only by exposure to sunlight but also to dim, diffused daylight.” However, these solar panels were very inefficient, especially compared to coal-fired power plants. In 1939, Russell Ohl created the solar cell design that is used in many modern solar panels. He patented his design in 1941. In 1954, this design was first used by Bell Labs to create the first commercially viable silicon solar cell. In 1957, Mohamed M. Atalla developed the process of silicon surface passivation by thermal oxidation at Bell Labs. The surface passivation process has since been critical to solar cell efficiency.
Solar cell panel, solar electric panel, photo-voltaic (PV) module or just solar panel is an assembly of photo-voltaic cells mounted in a framework for installation. Solar panels use sunlight as a source of energy to generate direct current electricity. A collection of PV modules is called a PV panel, and a system of PV panels is called an array. Arrays of a photovoltaic system supply solar electricity to electrical equipment. Again, the life span of the panels is around 30 years maximum, and it would be cheaper to discard than to recycle, leaving once again the problem of unsightly heaps of solar panels which are defunct and nobody knows what to do with them. In addition, understandably there will be variations in solar panel lifespans from manufacturer to manufacturer depending on the quality of build and materials. Typically, a solar panel’s design life is 25 to 30 years. What one needs to take into consideration when looking at this solar power system is the degradation rate of solar panels. This will determine the energy output as time goes on. Essentially, the degradation rate of your solar panel is the rate at which the solar panel loses efficiency over time. The typical degradation rate of quality-made solar panels is roughly 1% per year, although often it is even less than that. This means that in 25 years of use the majority of your solar panels will operate above 75% efficiency. As with any technology, he better you care for the equipment the longer you will enjoy the maximum benefits of the equipment. When it comes to solar power the solar panel is actually your most durable asset unlikely to suffer from massive degradation. Firstly, the best way to ensure solar power longevity is to use a reliable manufacturer and installer. Secondly, when your solar power system has been installed, make sure you inspect the system thoroughly upon installation as this is key to system longevity. Thirdly, there are three primary elements to a solar power system which you should regularly maintain. Inverter maintenance: the lifespan of an inverter is about 10 years – for most grid-tie inverters – which is why warranty extensions are often a good investment for inverters. Just check that the warranty covers the cost of the equipment and labour as well. Off-the-grid inverters tend to have a shorter lifespan of up to five years, which means you will likely replace the inverter a few times before replacing the solar panels. Annual preventative maintenance is a must to ensure consistently reliable output.
Battery maintenance: the highest cost of maintenance and replacement on a solar power system is the battery, particularly with off-the-grid systems as these cycle continuously. There are various types of batteries for solar power backup, the most common being VRLA or Lithium, with a design life of 5-10 or 15 years respectively.
Panel maintenance: This is largely overlooked, solar panel maintenance is a key factor to efficient output production. Dust and leaves can build up on panels, causing shady spots and effecting the panels ability to harness the sun’s energy. Temperature can also reduce a solar panel’s efficiency. Cooler panels produce better than hot panels and so some sites install sprinkler systems to keep their panels cool and clean.
As technology develops, so will the lifespan of the solar equipment. However, as it stands, solar panels are already advanced and offer consumers a great investment option. If your energy needs increase, you may need to invest in more solar panels at a later stage so ensure the inverter you buy now is scalable.
There are three types of solar panels. Each of these types of solar cells is made in a unique way and has a different aesthetic appearance. The types are: Mono crystalline, Poly crystalline and Thin-film solar panels. CdTe and CIGS solar cells contain cadmium, a toxic heavy metal with a tendency to accumulate in the food chain. Solder used in installation also contains lead. Leftover PV panels can contaminate soil, as it happened in 2013 when US-based Solyndra solar farm bankrupted leaving broken panels on site. IRENA 2016 study estimated the amount of PV waste at 78 millions tons by 2050. Most parts of a solar module can be recycled including up to 95% of certain semiconductor materials or the glass as well as large amounts of ferrous and non-ferrous metals. Some private companies and non-profit organizations are currently engaged in take-back and recycling operations for end-of-life modules. EU law requires manufacturers to ensure their solar panels are recycled properly. Similar legislation is underway in Japan, India, and Australia.
A 2021 study by Harvard Business Review indicates that by 2035 the discarded panels will outweigh new units by a factor of 2.56 and cost of recycling a single PV panel by then will reach $20-30, which would increase the LCOE of PV by a factor 4. Analyzing the US market, where no EU-like legislation exists as of 2021, HBR noted that with the cost of sending it to a landfill being just $1-2 there’s a significant financial incentive to either discard the decommissioned panels or send them to for low-tech disassembly in low-income countries with much of the toxic elements ending up released to the environment, while a mandatory recycling legislation would be necessary. Recycling possibilities depend on the kind of technology used in the modules. Silicon based modules: aluminum frames and junction boxes are dismantled manually at the beginning of the process. The module is then crushed in a mill and the different fractions are separated – glass, plastics and metals. It is possible to recover more than 80% of the incoming weight. This process can be performed by flat glass recyclers since morphology and composition of a PV module is similar to those flat glasses used in the building and automotive industry. The recovered glass, for example, is readily accepted by the glass foam and glass insulation industry.
Non-silicon based modules: they require specific recycling technologies such as the use of chemical baths in order to separate the different semiconductor materials. For cadmium telluride modules, the recycling process begins by crushing the module and subsequently separating the different fractions. This recycling process is designed to recover up to 90% of the glass and 95% of the semiconductor materials contained. Some commercial-scale recycling facilities have been created in recent years by private companies. For aluminum flat plate reflector: the trendiness of the reflectors has been brought up by fabricating them using a thin layer (around 0.016 mm to 0.024 mm) of aluminum coating present inside the non-recycled plastic food packages. Since 2010, there is an annual European conference bringing together manufacturers, recyclers and researchers to look at the future of PV module recycling.[28]
Another area of major concern is that of construction of mega hydroelectric dams. like the Aswan Dam[29] and presently the Grand Ethiopian Renaissance Dam in Ethiopia[30]. Apart from creating geopolitical tensions, between Ethiopia, Sudan and Egypt[31], for the use of the waters of the ancient River Nile, the construction of these dams has created great environmental impact in northeastern Africa, removing the natural habitat of hundreds of species of wildlife, as well as flora. The deforestation caused by the rising water levels has an immeasurable effect on GHG. In the Indian peninsula, in the foothills of the Himalayas[32], the Indian Government were building huge hydroelectric dams against the wise counsel of environmentalists. Around 125 people were missing in northern India after a Himalayan glacier broke and swept away a hydroelectric dam, with floods forcing the evacuation of villages downstream. The project was doomed to failure from the beginning, with the mighty Himalayas’ having their final say in the matter. Earlier this year, the half-built mega-dam, hydroelectric projects were totally washed away by flash floods in the region. A wall of dust, rock and water hit as an avalanche roared down the Rishiganga[33] valley deep in the mountains of Uttarakhand, a witness said.[34]
The Bottom Line is that while humanoids are clamouring for “Green Energy” to halt the emission of Green House Gases” and end the use of fossil fuels, it is ironic that humanity is exchanging one evil with a another, while, the serious human and environmental impacts are totally unknown. The new ultra-luxury Mercedes Maybach electric[35] concept vehicle designed as a collaboration between was announced this week by the Mercedes Chief Design Officer Gorden Wagener[36] and fashion designer Virgil Abloh[37] debuts on December 01st to mark the 100th Anniversary of the Maybach[38]. It is little known if the rich and famous driving this high tech, AI facilitated electric vehicle will cause any health problems in humans and their pregnant spouses. This possibility is akin to the use of radioactivity, when first discovered on November 8, 1895, by physicist Wilhelm Conrad Röntgen[39] (1845-1923) becomes the first person to observe X-rays, a significant scientific advancement that would ultimately benefit a variety of fields, most of all medicine, by making the invisible visible. This new discovery eventually led to cancers. Similarly, the “quest for Green energy”, like the Holy Grail by the Crusaders is fraught with unforeseen dangers, which may outweigh the benefits and plough humanity backs as did by the use of nuclear power, with the Chernobyl meltdown[40] in former Soviet Union and more recently in 2011 Fukushima Daiichi nuclear disaster in Japan[41]. Furthermore, the impact on environmental and human health of discarded lithium, batteries[42] will be immeasurable from mining of rare minerals, processing, recycling, acquisition and manufacturing of the end product used for the generation of “Green Energy”
More importantly, the access to critical minerals will serve to ensure that any country is able to achieve a future that is not entirely dependent on oil, as so many of these minerals are a prerequisite to EV and battery production. This could lead to a novel era of colonisation and domination by super powers, of poor countries, in order to secure a supply of these material for a sustained availability of “Green Energy”. In order to facilitate the transition to a more energy efficient and secure transportation system, the world’s vulnerability to critical mineral supply disruptions must be reduced. Increasing domestic exploration, production of critical minerals, promoting recycling and reprocessing are necessary to ensure automakers and technology developers have the resources they need to develop an advanced fuel vehicle transportation system. In this manner, the environmental impact might be mitigated, but these measures adopted by countries will definitely cause economic crises and global conflict, as it did between the British Raj[43] from 1858 to 1947 and its colonies, such as India[44] with the imposition of salt tax and destroying the Indian cotton industry[45], in the 19th and 20th centuries. The transition to a new energy system is often understood as a conflict between incumbent fossil fuel firms and proponents of climate action. As existential as this conflict is, battles between competing visions of a low-carbon world are intensifying – and they will become increasingly central to politics across the world. These competing visions reflect the reality that there are multiple paths to rapid energy transformation in the 21st century so as to contain global warming. The question is not whether to undergo a transformation from one energy system to another, but to ensure that humanity is not mortgaging its soul to “Green Energy”, with unimaginable challenges in the future.
References:
[1] https://en.wikipedia.org/wiki/Industrial_Revolution
[2] https://www.bing.com/search?FORM=TLCCLE&q=Industrial+Revolution%2c+The+steam+engine+is+invented&filters=lite:%22.VGltZWxpbmVQb2xlXjJBNzg4RTQzNTlGOUIzRjcyMEM3MjZDOUQwNUJEMDIyXl5eXklzTWluaXxUaW1lbGluZU1haW5eZTIzZmY2OTgtYzAwZC05MjBiLWVkNTktNGUwMDBiOWZkNTRlXzRBOEVBN0I5MERERkM1NENCQzVBRUExRTMwNEFGQ0E0Xl5eXjJBNzg4RTQzNTlGOUIzRjcyMEM3MjZDOUQwNUJEMDIyLEluZHVzdHJpYWwgUmV2b2x1dGlvbiwsLCwsMCxlMjNmZjY5OC1jMDBkLTkyMGItZWQ1OS00ZTAwMGI5ZmQ1NGU=%22+eventId:%22e23ff698-c00d-920b-ed59-4e000b9fd54e_4A8EA7B90DDFC54CBC5AEA1E304AFCA4%22+secq:%22Industrial%20Revolution%20timeline%22
[3] https://en.wikipedia.org/wiki/Fossil_fuel
[4] https://www.bing.com/search?q=discovery+of+oil+in+the+Arabian+Peninsula&aqs=edge..69i57.33157684j0j1&pglt=43&FORM=ANNTA1&PC=U531#:~:text=Image%3A%20amazon.com-,On%2031%20May%201932%2C%20the%20SOCAL%20subsidiary%2C%20the%20Bahrain%20Petroleum%20Company%20(BAPCO)%20struck%20oil%20in%20Bahrain.%20The%20discovery%20brought%20fresh%20impetus%20to%20the%20search%20for%20oil%20on%20the%20Arabian%20peninsula,-.%20Negotiations%20for%20an
[5] https://www.historichotels.org/us/hotels-resorts/omni-shoreham-hotel-washington-dc/history.php
[6] https://en.wikipedia.org/wiki/Saud_of_Saudi_Arabia
[7] https://en.wikipedia.org/wiki/Aswan_Dam
[8] https://www.livescience.com/37360-abu-simbel.html#:~:text=The%20site%20of%20Abu%20Simbel%20is%20one%20of,for%20the%20Aswan%20High%20Dam%20in%20the%201960s
[9] https://en.wikipedia.org/wiki/Industry_4.0
[10] https://www.ibm.com/cloud/learn/what-is-artificial-intelligence
[11] https://www.twi-global.com/technical-knowledge/faqs/what-is-green-energy
[12] https://en.wikipedia.org/wiki/Greenhouse_gas
[13] https://indusedu.org/pdfs/IJREISS/IJREISS_3686_45656.pdf
[14] https://www.power-technology.com/features/feature-biggest-wind-farms-in-the-world-texas/
[15] https://www.solarreviews.com/blog/what-is-a-solar-farm-do-i-need-one#:~:text=Solar%20farms%20are%20large-scale%2C%20ground-mounted%20solar%20installations.%20They,%E2%80%98solar%20parks%2C%E2%80%99%20%E2%80%98solar%20plants%E2%80%99%20and%20%E2%80%98solar%20power%20stations.%E2%80%99
[16] https://www.aljazeera.com/news/2021/2/8/china-to-build-the-worlds-biggest-dam-on-sacred-tibetan-river#:~:text=The%20mega-dam%20could%20produce%20as%20much%20as%20three,central%20China%20is%20currently%20the%20world%E2%80%99s%20biggest%20dam.
[17] https://en.wikipedia.org/wiki/Yarlung_Tsangpo_River
[18] https://whatis.techtarget.com/definition/photovoltaic-cell-PV-Cell#:~:text=A%20photovoltaic%20cell%20%28PV%20cell%29%20is%20a%20specialized,%28IR%29%20or%20ultraviolet%20%28UV%29%20radiation%20into%20DC%20electricity.
[19] https://www.mercedes-benz.com/en/vehicles/passenger-cars/eqs/eqs/
[20] https://en.wikipedia.org/wiki/Lithium
[21] https://en.wikipedia.org/wiki/Copper
[22] http://energyfuse.org/electric-vehicle-production-critical-minerals-supply/#:~:text=Every%20EV%20needs%20magnets%2C%20microprocessors%2C%20and%20batteries%2C%20all,and%20the%20rare%20earth%20elements%20dysprosium%20and%20neodymium.
[24] https://www.nationalgeographic.com/environment/article/fukushima-tragic-legacy-radioactive-soil#:~:text=In%202020%2C%20the%20Japanese%20government%20lifted%20bans%20on,some%20of%20the%20reactors%20there%20are%20still%20leaking.
[25] https://en.wikipedia.org/wiki/Chuquicamata
[26] https://en.wikipedia.org/wiki/Salar_de_Atacama
[27] http://www.aps.org/publications/apsnews/200904/physicshistory.cfm
[28] https://en.wikipedia.org/wiki/Solar_cell_panel#:~:text=%22First%20Breakthrough%20In%20Solar%20Photovoltaic%20Module%20Recycling%2C%20Experts%20Say%22.%20European%20Photovoltaic%20Industry%20Association.%20Archived%20from%20the%20original%20on%2012%20May%202013.
[29] https://www.bing.com/search?q=Abusinble+Temple+and+Aswan+Dam&cvid=f493f21aa9674eb2a6a6a57bd780f87e&aqs=edge..69i57.13156j0j1&pglt=43&FORM=ANNTA1&PC=U531#:~:text=The%20Aswan%20dam%20was%20crucial%20to%20Egypt%E2%80%99s%20economic%20development%20but%20the%20area%20marked%20for%20inundation%20was%20also%20the%20location%20of
[30] https://www.water-technology.net/projects/grand-ethiopian-renaissance-dam-africa/#:~:text=The%20Grand%20Ethiopian%20Renaissance%20Dam%20%28GERD%29%2C%20formerly%20known,is%20owned%20by%20Ethiopian%20Electric%20Power%20Corporation%20%28EEPCO%29.
[31] https://www.dailymaverick.co.za/article/2021-03-23-grand-ethiopian-renaissance-dam-geopolitical-tension-escalates-the-potential-for-a-war-in-horn-of-africa/
[32] https://en.wikipedia.org/wiki/Himalayas
[33] https://en.wikipedia.org/wiki/Rishiganga
[34] https://nationalpost.com/news/world/himalayan-glacier-sweeps-away-hydroelectric-damn-in-northern-india-as-officials-fear-125-still-missing#:~:text=A%20wall%20of%20dust%2C%20rock%20and%20water%20hit%20as%20an%20avalanche%20roared%20down%20the%20Rishiganga%20valley%20deep%20in%20the%20mountains%20of%20Uttarakhand%2C%20a%20witness%20said.
[35] https://www.carscoops.com/2021/10/new-electric-mercedes-maybach-concept-announced-debuts-december-1st/
[36] https://www.mercedes-benz.com/en/design/team-and-studios/gorden-wagener-vice-president-design-daimler-ag/
[37] https://www.yahoo.com/lifestyle/virgil-abloh-design-mercedes-maybach-134859499.html
[38] https://www.topgearmag.in/mercedes-maybach-celebrates-100-years-with-the-edition-100-s-class/#:~:text=This%20year%20marks%20the%20100-year%20anniversary%20of%20the,on%20sale%20in%20the%20first%20half%20of%202022.
[39] https://en.wikipedia.org/wiki/Wilhelm_R%C3%B6ntgen
[40] https://en.wikipedia.org/wiki/Chernobyl_disaster#:~:text=The%20Chernobyl%20disaster,the%20other%20being
[41] https://en.wikipedia.org/wiki/Chernobyl_disaster#:~:text=the%202011%20Fukushima%20Daiichi%20nuclear%20disaster%20in%20Japan
[42] https://www.mewsie.org/textbook/potential-environmental-and-human-health-impacts-of-rechargeable-lithium-ion-and-lithium-polymer-batteries-in-discarded-cellular-phones/
[43] https://en.wikipedia.org/wiki/British_Raj
[44] https://www.primidi.com/history_of_the_british_salt_tax_in_india/taxation_of_salt_in_india/salt_laws
[45] http://indiafacts.org/british-destroyed-indian-textile-industry/
______________________________________________
Professor G. Hoosen M. Vawda (Bsc; MBChB; PhD.Wits) is a member of the TRANSCEND Network for Peace Development Environment.
Director: Glastonbury Medical Research Centre; Community Health and Indigent Programme Services; Body Donor Foundation SA.
Principal Investigator: Multinational Clinical Trials
Consultant: Medical and General Research Ethics; Internal Medicine and Clinical Psychiatry:UKZN, Nelson R. Mandela School of Medicine
Executive Member: Inter Religious Council KZN SA
Public Liaison: Medical Misadventures
Activism: Justice for All
Email: vawda@ukzn.ac.za
Tags: Energy, Renewable Energy, Sustainable energy
This article originally appeared on Transcend Media Service (TMS) on 18 Oct 2021.
Anticopyright: Editorials and articles originated on TMS may be freely reprinted, disseminated, translated and used as background material, provided an acknowledgement and link to the source, TMS: “Green Energy”: Are Humanoids Safe?, is included. Thank you.
If you enjoyed this article, please donate to TMS to join the growing list of TMS Supporters.
This work is licensed under a CC BY-NC 4.0 License.